Primitive organisms have no nervous systems.
However they have a lot of the biochemical machinery for synaptic communication.
It is theorized that synapses began as a damage control response to membrane rupture in single celled organisms. When a membrane ruptures, calcium rushes into the cell, which is toxic because it forms insoluble precipitates with phosphate. So two things happened: 1) calcium triggered a contractile response around the rupture using actin and myosin, and 2) calcium caused exocytosis to provide new membrane material to heal the rupture. These two mechanisms are what we understand today as neurotransmitter release from actively transported vesicles that fuse with the synaptic membrane upon nerve activation.
However the picture gets a lot more interesting when you start looking at action potentials. Why action potentials? Why is it necessary for nerves to "fire"? The earliest organisms had no action potentials, they communicated with "gap junctions" which are just ion channels from one cell to the next. So instead of firing, they simply communicated a value, an average level of activity. Some plants have action potentials, and some early eukaryotes don't. However in all cases the basic biochemical machinery is present.
One theory holds that action potentials began to increase the speed of communication in long nerves. For example worms are long and skinny, but they have a brain at one end, and to get the information from one end to the other requires speed.
But it turns out there's something more interesting going on. Finite dimensional linear systems are incapable of chaotic behavior. They can't take a small signal and amplify it network wide. So, while they might be able to discriminate a hazardous condition, they can't act on it in a timely and focused manner. An example is provided by the withdrawal reflex in a jellyfish. It takes over the whole brain, resulting in ink spraying and a coordinated movement of the tentacles in a direction away from the hazard. Studies with artificial neural nets have confirmed the importance of chaotic dynamics in these kinds of global reflexes.
link.aps.org
Action potentials are thought to have evolved during the Ediacaran period about 600 million years ago. Sponges (porifera) have no action potentials, although they do have secretory (synaptic-like) systems. Jellyfish have action potentials, but they transmit across gap junctions rather than synapses. The first actual neuron appears late in the coelenterates, which have nerve "nets" (as distinct from brains). By the time we get to bilateria we see full neurons. The primitive ones modulate their behavior by altering ion channel properties, rather than by synaptic variation. The action potentials are slow, there's no myelin. For instance the squid (loligo) giant axon is unmyelinated, whereas the earthworm giant median fibers are myelinated. We first see real myelin in the placoderms (with hinged jaws) about 425 million years ago, although the chemical prerequisites are in place 50 million years earlier.
www.ncbi.nlm.nih.gov
There is a theory that says myelin originated from a random injection of DNA by a virus.
www.biorxiv.org
Following comes the development of nervous organelles, like eyes. Photoreceptor cells exist early on, but they don't get connected to the full nervous system till planaria. The first known actual eye was in a trilobite.
www.ncbi.nlm.nih.gov
www.nature.com
Eyes are interesting because they show us how nature builds "systems" with a wide variety of neural types and behaviors. In the human eye the first layers are devoted to gain control while the later layers encode color and motion. The earliest central visual structure is involved with visual attention and focusing the eyes on interesting stimuli, and we don't really see full criticality till we get to the visual cortex.
news.uark.edu
pubmed.ncbi.nlm.nih.gov
However they have a lot of the biochemical machinery for synaptic communication.
It is theorized that synapses began as a damage control response to membrane rupture in single celled organisms. When a membrane ruptures, calcium rushes into the cell, which is toxic because it forms insoluble precipitates with phosphate. So two things happened: 1) calcium triggered a contractile response around the rupture using actin and myosin, and 2) calcium caused exocytosis to provide new membrane material to heal the rupture. These two mechanisms are what we understand today as neurotransmitter release from actively transported vesicles that fuse with the synaptic membrane upon nerve activation.
However the picture gets a lot more interesting when you start looking at action potentials. Why action potentials? Why is it necessary for nerves to "fire"? The earliest organisms had no action potentials, they communicated with "gap junctions" which are just ion channels from one cell to the next. So instead of firing, they simply communicated a value, an average level of activity. Some plants have action potentials, and some early eukaryotes don't. However in all cases the basic biochemical machinery is present.
One theory holds that action potentials began to increase the speed of communication in long nerves. For example worms are long and skinny, but they have a brain at one end, and to get the information from one end to the other requires speed.
But it turns out there's something more interesting going on. Finite dimensional linear systems are incapable of chaotic behavior. They can't take a small signal and amplify it network wide. So, while they might be able to discriminate a hazardous condition, they can't act on it in a timely and focused manner. An example is provided by the withdrawal reflex in a jellyfish. It takes over the whole brain, resulting in ink spraying and a coordinated movement of the tentacles in a direction away from the hazard. Studies with artificial neural nets have confirmed the importance of chaotic dynamics in these kinds of global reflexes.
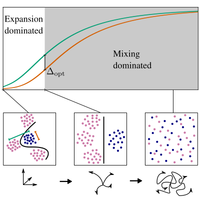
Transient Chaotic Dimensionality Expansion by Recurrent Networks
A framework for comparing models of neural networks provides insight into how the brain can support powerful computations despite its strongly chaotic electrical activity.
Action potentials are thought to have evolved during the Ediacaran period about 600 million years ago. Sponges (porifera) have no action potentials, although they do have secretory (synaptic-like) systems. Jellyfish have action potentials, but they transmit across gap junctions rather than synapses. The first actual neuron appears late in the coelenterates, which have nerve "nets" (as distinct from brains). By the time we get to bilateria we see full neurons. The primitive ones modulate their behavior by altering ion channel properties, rather than by synaptic variation. The action potentials are slow, there's no myelin. For instance the squid (loligo) giant axon is unmyelinated, whereas the earthworm giant median fibers are myelinated. We first see real myelin in the placoderms (with hinged jaws) about 425 million years ago, although the chemical prerequisites are in place 50 million years earlier.
Proposed evolutionary changes in the role of myelin - PMC
Myelin is the multi-layered lipid sheet periodically wrapped around neuronal axons. It is most frequently found in vertebrates. Myelin allows for saltatory action potential (AP) conduction along axons. During this form of conduction, the AP travels ...

There is a theory that says myelin originated from a random injection of DNA by a virus.

A retroviral origin of vertebrate myelin
Myelin, the insulating sheath that surrounds neuronal axons, is produced by oligodendrocytes in the central nervous system (CNS). This evolutionary innovation, which first appears in jawed vertebrates, enabled rapid transmission of nerve impulses, more complex brains and greater morphological...
Following comes the development of nervous organelles, like eyes. Photoreceptor cells exist early on, but they don't get connected to the full nervous system till planaria. The first known actual eye was in a trilobite.
Eye evolution and its functional basis - PMC
Eye evolution is driven by the evolution of visually guided behavior. Accumulation of gradually more demanding behaviors have continuously increased the performance requirements on the photoreceptor organs. Starting with nondirectional ...

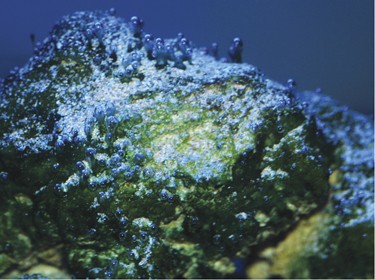
The evolution of eyes: major steps. The Keeler lecture 2017: centenary of Keeler Ltd - Eye
Ocular evolution is an immense topic, and I do not expect to cover all the details of this process in this manuscript. I will present some concepts about some of the major steps in the evolutionary process to stimulate your thinking about this interesting and complex topic. In the prebiotic...

Eyes are interesting because they show us how nature builds "systems" with a wide variety of neural types and behaviors. In the human eye the first layers are devoted to gain control while the later layers encode color and motion. The earliest central visual structure is involved with visual attention and focusing the eyes on interesting stimuli, and we don't really see full criticality till we get to the visual cortex.
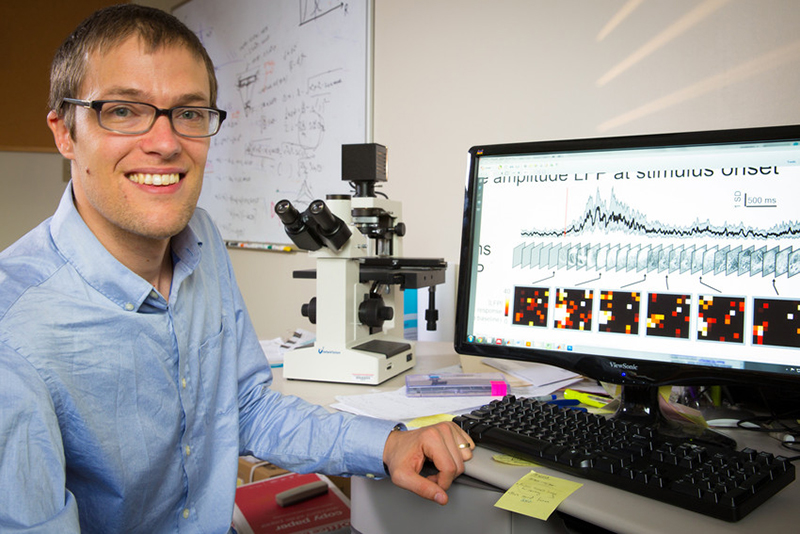
On the Brink of Chaos: Physicists Find Phase Transition in Visual Cortex
Physicists have discovered that the visual cortex spontaneously returns the brain to its optimal function during intense visual input.
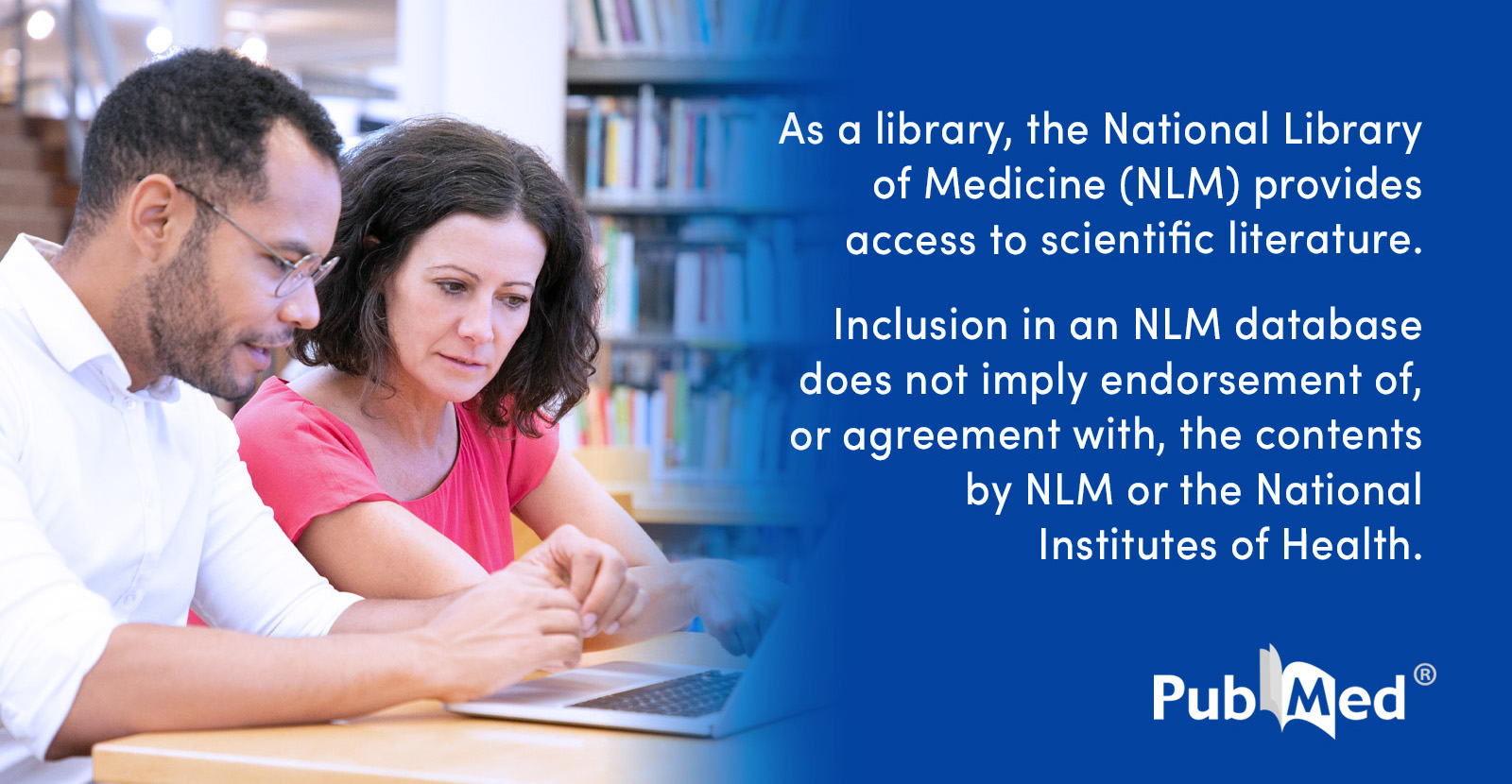
Chaos and synchrony in a model of a hypercolumn in visual cortex - PubMed
Neurons in cortical slices emit spikes or bursts of spikes regularly in response to a suprathreshold current injection. This behavior is in marked contrast to the behavior of cortical neurons in vivo, whose response to electrical or sensory input displays a strong degree of irregularity...
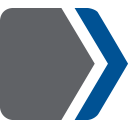